The value of Fulvic Acid in hydroponics
Fulvic and humic acids have been studied for decades and used extensively in the soil and hydroponic growing industries. I previously talked about the use of humic acid in hydroponics and the way in which it can improve crop results. In that post, we talked about how humic acids can improve nutrient chelation and how this can lead to improvements in yields depending on the origin and properties of the humic substances used. In this post, we are going to take a look specifically at fulvic acid substances, which are a smaller family that has potentially more valuable uses in the hydroponic space. We will start by discussing what differentiates fulvic and humic acids and what the current peer-reviewed evidence around fulvic acids tells us.
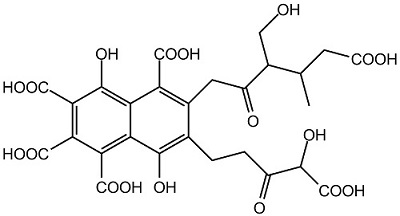
Fulvic acids are not chemically pure substances, but a group of chemicals that result from the decomposition of organic matter. This process generates both humic and fulvic acids. However, fulvic acids are different from humic acids in mainly two ways. The first is that fulvic acids are soluble at both acid and alkaline pH values, and the second, is that fulvic acids generally have much lower molecular weights. Fulvic acids are therefore more soluble and are more easily accessible to plants compared to humic acids, which have much larger molecular weights. But why should we use them in hydroponics and exactly how?
Sadly, not many publications have tackled the use of fulvic acid in crops specifically. One of the few examples of reviews that touch on the matter is this paper, which covers most of the literature around fulvic acids before 2014. I also did a literature review myself, trying to find articles in which the fulvic acid source, application type and rate, and the results against a control without fulvic acid were clearly explained. The table below shows you the results of my search, I was able to find 10 papers overall, with a mix of root and foliar applications of fulvic acid, with a range of application rates and plant species. Almost all of these papers found positive results from the use of fulvic acid, except two papers that found either no effect or mixed results from their use.
The range of application depends substantially on the application type. Most papers that tackled foliar applications chose application rates in the 1-3g/L range, while papers that tackled root applications generally stayed in the 25-150ppm range. This is normal since foliars are generally much more concentrated than root applications. Both types of applications have different effects. Root applications are going to exercise an additional strong nutrient chelating role, while foliar applications are more likely to exert a hormonal role. A study around genetically modified tomato plants showed that plants engineered to be insensitive to IAA were also unable to respond to fulvic acid, hinting at the fact that fulvic acid has an auxin-like effect in plants.
Ref | Application Type | Crop | Application (ppm) | Effect |
1 | Foliar | Tomato | 800-1100 | yield+ number+ cracking- |
2 | Root | Cucumber | 100-300 | growth+ |
3 | Foliar | Grapevines | 500 | yield+ growth+ quality+ |
4 | Root | Pepper | 25 | quality+ |
5 | Foliar | Wheat | 500-1000 | no effect |
6 | Root | Impatiens | 40 | yield+ flowering+ |
7 | Foliar | Faba Beans | 1500-3000 | yield+ |
8 | Root | Tomato | 15-30 | mixed |
9 | Root | Okra | 1500-3000 | yield+ quality+ |
10 | Root | Potato | 150 | yield+ |
The effects seem to be quite positive overall, with increases in yield, quality, and flower numbers across the board. The studies above that investigated nutrient transport also showed substantial benefits when root applications of fulvic acid were used. Plants grown in a Hoagland solution showed better nutrient transport when fulvic acid substances were used in the nutrient solution. This is possibly both due to their ability to chelate micronutrients and their ability to provide an additional pH buffer at the region of interest in hydroponics (5.5-6.5). This study, shows how fulvic acid substances can have pKa values in this precise region, although their still relatively large molar mass implies that they will contribute marginally to buffering capacity, especially if used only in <100 ppm concentrations.
Fulvic acids also seem to be synergistic with several other biostimulants in the studies showed above. When tests were done with humic acids or other biostimulants, the effect of the combination is usually better than the effect of either part on its own. This means the fulvic acid might not only be a good addition on its own, but it might also contribute significantly to enhance the effect of other biostimulants used.
It is however important to note that fulvic acids do have negative effects when used in excess, reason why their application rates need to be carefully controlled. Using too much can lead to drops in yields and quality along with slower growth. If you want to start using them, it is, therefore, wise to start at the lower range of the application rates shown above and climb up as you gauge the effects. It is also important to note that – as humic acids – different sources of fulvic acid might have different effects, as the actual molecules that make up the substance will change.
A big advantage of the use of fulvic acids in hydroponics is also that their solubility is quite high, so the risk of clogging or damaging equipment is low. This is a significant advantage over humic acids, which have lower solubility and can cause problems because of this in hydroponics culture, especially if there are drops in the pH. In hydroponics, fulvic acids can also lead to additional solution stability, especially in recirculating systems, where the destruction of heavy metal chelates as a function of time can become a bigger risk.
All in all, fulvic acids represent a relatively cheap addition to a hydroponic regime that has limited risk and a lot of potential upsides. Literature research shows us that low rate applications, if anything, might just have no effect, so the risk of damage to a hydroponic crop by trying fulvic acid applications is low. The synergistic effects shown by fulvic acid are also interesting since this means that they might make other additives you are currently using even more potent. When looking for fulvic acids, make sure you check for high solubility, solubility in low and high pH, and a source that matches the sources used in the literature results you’re interested in reproducing.