Five tips to successfully manage your nutrient solution in a recirculating hydroponic setup
Although a significant portion of hydroponic growers use run-to-waste setups – where the nutrient solution is ran through plants and then lost – the industry is now moving towards the implementation of recirculating hydroponic systems in order to reduce both water usage and the unnecessary dumping of fertilizers into sewage systems. A recirculating setup has many advantages and can provide better yields than run-to-waste setups, provided the solution is properly managed and changed through the growing cycle. In this post I’m going to talk about five tips that will help you successfully manage your nutrient solution when using this type of system.
Ensure the volume of the reservoir is at least 10x the volume necessary for a single irrigation. The total volume of a reservoir is key in a recirculating setup because you want the bulk of the solution to be unaffected by whatever nutritional changes are caused by the plants during each feeding. This means that you want most of the solution to be inside your tanks and not inside the media when every irrigation is done. A simple rule of thumb is to make the volume of your initial reservoir at least 10x the volume that it would take to carry out a single irrigation of your entire crop. If you do this the water and nutrient absorption effects will happen slowly and will give you time to manage your solution without any harm coming to the plants.
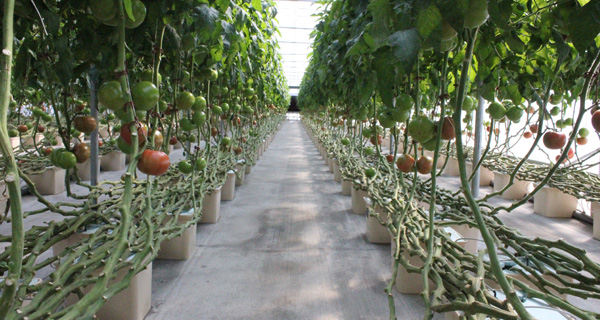
Circulate your solution until your pH and EC are constant. After an irrigation cycle starts, the solution will first mix with the remnants of the last irrigation cycle within the media, which will make the pH and EC of the return different from those of the main tank. In order to ensure that the plant’s root system is being subjected to the desired nutrient concentrations, make sure you carry out the recirculating process until the EC and pH of the tank remains constant and matches the return pH and EC. Once this happens you know that the conditions within the media have now been equalized with the larger body of solution and you can stop the irrigation process. Constant monitoring of the pH and EC within the tank are therefore necessary within this type of setup.
Add water and not nutrients when the EC increases with every irrigation. In a normal recirculating setup the EC of the solution in the main tank will tend to increase with every irrigation while the total volume of the solution will decrease. This happens because healthy plants always absorb more water than nutrients, which means any measure that’s proportional to concentration – such as the EC – will tend to increase as the amount of water goes down. You want to add enough water to bring the EC down to the desired EC but you do not want to add nutrients with this water and this would increase the EC or contribute to nutrient imbalances within the solution. Note that you will need to add less water than the amount that was absorbed by the plants, because the plants also take some nutrients with them, meaning that the amount of water needed to reestablish the EC to what it was before will be lower. If an initial solution has 1000 gallons, the volume might go down to 950 gallons on the first irrigation but you might only need to add 20 gallons to bring it back to the original EC, making the total in the end around 970 gallons. Make sure the pH of the tank is also corrected after every irrigation and water addition.
Replenish water with nutrients when volume is down 40%, use this as an opportunity to shift the solution. As discussed in the last tip, the volume of solution will go down with time, even if some water is added to return to the original EC. At some point more than 40% of the volume will have been spent and it is at this point where you should fill the tank back to its full volume with water plus nutrients. You can also use this opportunity to change the nutrient ratios and skew them in the direction that you want your plants to follow nutritionally. For example in a flowering crop it is common to increase the amount of potassium during the blooming stages of the plant, so this can be done as nutrient solution is replenished after it’s consumed by the plants. Note that this process cannot be carried out indefinitely because both nutrient imbalances and plant exudates will accumulate within the main solution. Most recirculating crops will fully change the solution every 3-4 weeks to avoid these problems although the life of the solution can be extended further when chemical analysis is done – to customize nutrient replenishing – and adequate filtering is implemented to remove substances contributed by plants.
Add in-line UV filters and carbon filters. It is fundamental to ensure no microorganisms contaminate your nutrient solution. For this reason, online UV-filters are necessary to keep the nutrient solution as sterile as possible. Carbon filters are also very useful as they remove plant exudates that can contaminate the solution and cause problems within the crop itself. Many of these exudates are food for microorganisms, others are plant hormones that might cause unwanted responses in the plants. However both carbon filtration and UV filters can cause some issues – such as the destruction and adsorption of heavy metal chelates – so it is important to use chelates that are more resistant to UV and have less affinity for carbon filters to alleviate these problems.
There is certainly a lot more to the management of recirculating hydroponic solution than what I have detailed above, it is important to note that some of these tips are simplifications and much better tailor-made solutions are possible with a proper analysis of each situation. These are just some simple tips to hopefully make your change towards the use of recirculating systems a lot easier and should greatly increase your chances of success in the world of recirculating hydroponic setups.