Using calcium sulfate in hydroponics
Calcium is a very important element in plant nutrition and can be supplied to plants through a wide variety of different salts. However, only a handful of these resources are significantly water soluble, usually narrowing the choice of calcium to either calcium nitrate, calcium chloride or more elaborate sources, such as calcium EDTA. Today I am going to talk about a less commonly used resource in hydroponics – calcium sulfate – which can fill a very important gap in calcium supplementation in hydroponic crops, particularly when Ca nutrition wants to be addressed as independently as possible and the addition of substances that interact heavily with plants wants to be avoided.
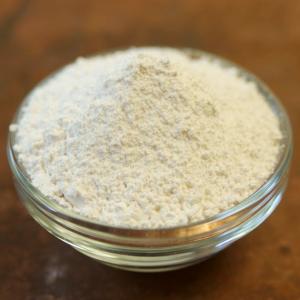
There are some important reasons why you don’t hear too much about calcium sulfate in hydroponics. Some websites actually recommend heavily against using this substance in hydroponic nutrient solutions. Why is this the case? The core issue is calcium sulfate’s solubility, with this substance traditionally considered “insoluble” in chemistry. However all substances are soluble to one or another degree – even if to an extremely small degree – but calcium sulfate is actually at the very border of what is considered a soluble substance in regular aqueous chemistry.
At 20C (68F), calcium sulfate dihydrate – the form most commonly available – has a solubility of around 2.4 g/L. In practice this means that you can have up to around 550 ppm of Ca in solution from calcium sulfate dihydrate before you observe any precipitation happening. This is way more than the normal 150-250 ppm of Ca that are used in final hydroponic nutrient solutions that are fed to plants. You could supply the entire plant requirement for calcium using calcium sulfate without ever observing any precipitate in solution. At the normal temperature range that hydroponic nutrient solutions are kept, the solubility of calcium sulfate is just not an issue. To add 10 ppm of Ca from calcium sulfate you need to add around 0.043g/L (0.163g/gal). You should however avoid using calcium sulfate for the preparation of solutions for foliar sprays as it will tend to form precipitates when the foliar spray dries on leaves, the leaves will then be covered with a thin film of gypsum, which is counterproductive.
Calcium sulfate has a great advantage over other ways to supplement calcium in that the anion in the salt – sulfate – does not contribute as significantly to plant nutrition. Other sources, such as calcium chloride or calcium nitrate, will add counter ions that will heavily interact with the plant in other ways, which might sometimes be an undesirable effect if all we want to address is the concentration of calcium ions. Other sources such as Ca EDTA might even add other cations – such as sodium – which we would generally want to avoid. Calcium sulfate will also have a negligible effect in the pH of the solution, unlike other substances – like calcium carbonate – which will have a significant effect in the pH of the solution.

A key consideration with calcium sulfate is also that its dissolution kinetics are slow. It takes a significant amount of time for a given amount of calcium sulfate to dissolve in water, even if the thermodynamics favor the dissolution of the salt at the temperature your water is at. For this reason it is very important to only use calcium sulfate sources that are extremely fine and are graded for irrigation. This is sometimes known as “solution grade” gypsum. I advice you get a small amount of the gypsum source you want to use and test how long it takes to dissolve 0.05g in one liter of water. This will give you an idea of how long you will need to wait to dissolve the calcium sulfate at the intended temperature. Constant agitation helps with this process.
An important caveat with calcium sulfate is that its relatively low solubility compared with other fertilizers means that it cannot be used to prepare concentrated nutrient solutions. This means that you will not be able to prepare a calcium sulfate stock solution or use calcium sulfate in the preparation of A and B solutions. As a matter of fact the formation of calcium sulfate is one of the main reasons why concentrated nutrient solutions usually come in two or more parts, to keep calcium and sulfate ions apart while they are in concentrated form. Calcium sulfate should only be added to the final nutrient solution and adequate considerations about temperature and dissolution time need to be taken into account.