A guide to different pH up options in hydroponics
When is pH up needed?
The control of pH in hydroponics is critical. Most commonly, we need to decrease the pH of our solutions as most nutrients will initially be at a higher than desired pH. This is especially true when tap water or silicates are used, as both of these inputs will increase the overall pH of hydroponic nutrients after they are prepared. In recirculating systems, pH will also tend to drift up due to the charge imbalance created by the high active uptake of nitrate ions carried out by most plant species. For a discussion on pH down options, please read my previous post on this topic.
However, there are certain circumstances where the pH of hydroponic solutions needs to be increased. This can happen when tap water or silicates are not used or when plants decrease pH due to an aggressive uptake of some cations. Plants like tomatoes can do this when grown in solutions with high potassium contributions, as they will actively uptake these nutrients to the point of changing pH balance. Excess ammonium can be another common cause for pH decreases in hydroponic solutions that require the use of pH up solutions.
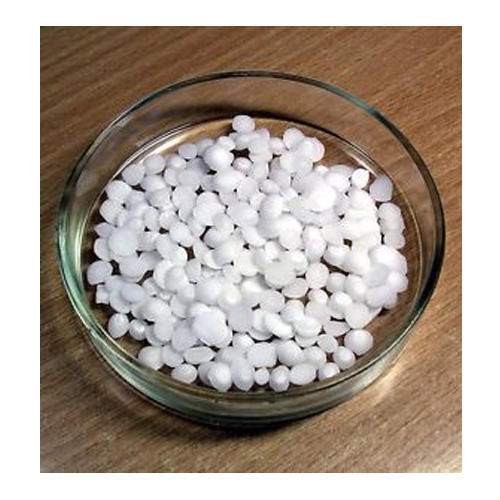
With this in mind, let’s discuss the pH up options that are available in hydroponics. I only considered substances that are soluble enough to create concentrated solutions, such that they can be used with injector systems.
pH up options
Sodium or potassium hydroxide (NaOH, KOH)
These are the strongest. They are low cost, can be used to prepare highly concentrated solutions and will increase the pH most effectively. They are however unstable as a function of time because they react with carbon dioxide from the air to form sodium or potassium carbonates. This means that their concentrated solutions need to be kept in airtight containers and that their basic power will decrease with time if this is not the case. Additionally, these hydroxides are extremely corrosive and their powder is an important health hazard. Dissolving them in water also generates very large amounts of heat – sometimes even boiling the water – which makes their usage more dangerous. Although desirable when basic power is the most important short term concern, I recommend to avoid them giving their PPE requirements and the lack of long term stability.
When these hydroxides are used, potassium hydroxide is the recommended form, as potassium hydroxide is both more basic and a plant nutrient, while excess sodium can cause problems with plant development. However, sodium hydroxide might be more desirable if it can be obtained at a particularly low price and small additions of sodium are not a concern.
Potassium silicate
This is a soluble form of silicon that is stable at high pH values. While solutions of potassium silicate by itself can be prepared and used as a pH up option, it is usually stabilized with a small addition of potassium hydroxide to take the pH of solutions to the 11-12 range. Potassium silicate contributes both potassium and silicon to hydroponic solutions – both important nutrients – and its use can be more beneficial than the use of pure potassium hydroxide. While silicates are less basic and more mass is required for the same pH buffering effect, the preparation and handling can often be much simpler than those of potassium hydroxide.
Note that potassium silicate solutions are also unstable when left in open air, as they will also react with atmospheric carbon dioxide to generate potassium carbonate. It is also worth noting that not all potassium silicates are the same, when looking for a highly soluble potassium silicate for hydroponics, make sure you get potassium silicates that have higher K/Si ratios. Usually ratios of at least 1.05 are required (make sure you convert both K and Si to their elemental forms, as most of these products report K as K2O and Si as SiO2).
Potassium carbonate (K2CO3)
This basic salt is stable in air, has less demanding PPE requirements and can also be used to prepare concentrated solutions (more than 1g of potassium carbonate can be dissolved per mL of water). Because of its lower basicity compared to potassium hydroxide, more of it also needs to be used to increase the pH of a hydroponic solution. However, solutions of it are stable, so there is no concern for their stability or changes to its basic power.
Another advantage given by potassium carbonate is that – contrary to the previous two examples – it does increase the buffering capacity of the solution against pH increases, due to the addition of carbonate to the solution. As carbon dioxide is lost to the air at the pH used in hydroponics, the pH of the solutions tends to drift up, this means that the carbonate addition makes the pH more stable in solutions where the pH is being constantly pushed down. This is all part of the carbonic acid/bicarbonate equilibrium, which also helps chemically buffer the solutions at the pH used in hydroponics.
Overall potassium carbonate is one of my favorite choices when there is a downward drift of pH in recirculating solutions.
Potassium phosphate (K3PO4)
Another weak base, potassium phosphate, can be used to prepare concentrated solutions and increase the pH in hydroponic solutions. While its solubility and basicity are lower than that of potassium carbonate, it does provide additional phosphorus that can buffer the pH of the solution. This happens because mono and dibasic phosphate ions are anions that be taken up by plants, therefore decreasing the pH. While phosphates can help chemically buffer the hydroponic solution against pH increases, for decreases the phosphate buffer is ineffective as the pKa of the relevant equilibrium is 7.2.
An issue with potassium phosphate is that it provides large contributions of K to solution. These potassium additions can be quite counter productive if the cause of the pH drift towards the downside is related to potassium uptake.
Potassium Citrate/Lactate/Acetate
Basic organic salts of potassium can also be used to increase the pH. These are all much weaker than even the carbonate and phosphate bases mentioned above and relatively large additions are required for even a moderate immediate effect in pH. However, since these anions are actively taken up by microbes, the microbial metabolism of these ions will create a longer term effect on pH. A moderate addition of potassium citrate can only cause a small increase of pH in the short term, with a larger increase happening during the following 24 hours.
A disadvantage is that these anions can also lead to explosions in bad microbe populations if the environment does not have an adequate microbial population. When these salts are used, adequate microbial inoculations need to be carried out to ensure that the microbes that will proliferate will not be pathogenic in nature.
Protein Hydrolysates
While hydrolysates themselves can have an acidic pH when put in solution, their microbial metabolism aggressively increases the pH of solutions in the medium term. This means that these hydrolysates should not be used for immediate pH adjusting, as they will tend to decrease pH further in the very short term, but they can be used as a more long term management option.
As with the above organic salts, their use also requires the presence of adequate microbial life. If you neglect to properly inoculate the media before their addition, then pathogens can also make use of these amino acids to proliferate.
Combinations are also possible
As with the case of pH down options, some of the best solutions for a problem come when several of the above solutions are combined. For example the use of potassium rich pH up solutions in microbe containing soilless media can often cause pH drift issues related with potassium to worsen. For this reason, it can be desirable in these cases to prepare pH up solutions that include protein. This means that you reduce the pH fast but then you have a residual effect from protein metabolism that helps you fight the pH increase as a function of time.
However not all pH up drifts are caused by potassium, as in the case of plants where pH up drift happens due to low nitrate uptake (for example some flowering plants that stop producing a lot of additional leaves during their flowering stage). In these cases potassium based pH up solutions cause no additional issues and combinations of potassium carbonate and potassium phosphate might be best.
Choose according to your goals
As in most cases, the best solution will depend on your circumstances. Think about whether you’re just adjusting the pH of your initial solutions or whether you need to compensate for a constant drift, whether microbial life is present and whether you’re concerned with the accumulation of any substances in a recirculating solution. Once you consider these factors and review the above solutions, you should be able to find the pH up solution that is better suited to your particular needs.
Are you using a pH up? Let us know why and which one you’re using in the comments below!