Factors limiting the life of a recirculating hydroponic nutrient solution
Hydroponic systems that use recirculating nutrient solutions can be more efficient in terms of water and nutrient usage. However, despite how good the management of a solution is, there are certain factors that will limit the time that a solution can be maintained without performing a full change of the entire recirculating nutrient solution within the system. By performing actions to attenuate some of these factors the life of the nutrient solution can be increased but trying to keep a nutrient solution endlessly is often impractical, both from a technical and economic perspective. In today’s post I will talk about the factors that limit the life of a recirculating nutrient solution and some of the actions that can be taken to increase the life of the solution.
Selective nutrient uptake. Plants will uptake some nutrients significantly faster than they do others. This will lead to a substantial accumulation of certain nutrients within the solution if nutrients are replenished to keep the EC of the solution constant at constant volume. Most commonly phosphorous will tend to accumulate within the solution. This is because plants will uptake this nutrient significantly slower than the others, while it will be replenished in full strength every time nutrients are added. This will tend to increase the ratio of phosphate to other nutrients, eventually causing phosphorous, calcium and magnesium issues within the solution. Micronutrients will also be replenished more than they are consumed and micros like Molybdenum and copper can dangerously accumulate in solutions that are kept for long periods of time (months).
The above is the main reason why nutrients are often replaced every 2-4 weeks in recirculating hydroponic setups. Chemical analysis can help expand this time – by allowing the grower to selectively replace only the things that have been taken – but this requires growers to have experience in the calculation and creation of nutrient formulations and to be able to effectively adapt the nutrients as required.
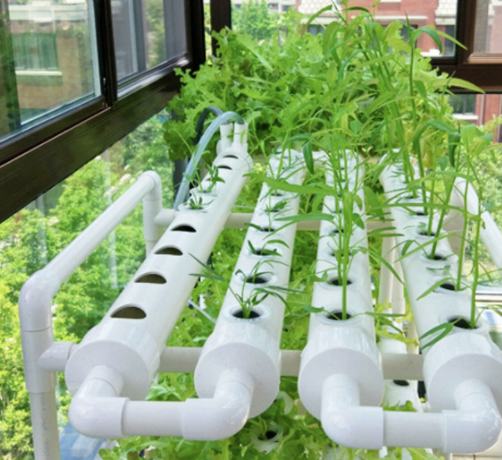
Contamination by pathogens. Nutrient solutions will get contaminated by external pathogens as they recirculate and come into contact with the media and the air. This contamination with pathogens might grow to the point that plants start developing disease, which can lead to substantial losses as diseases are spread incredibly efficiently within recirculating nutrient solutions. Potential solutions such as ozone and UV filtration can help eliminate the pathogens, but these oxidative actions will also destroy important aspects of the nutrient solution, such as the chelating agents that are used to wrap around heavy metal ions. This means that – as you destroy pathogens – you will lose heavy metal availability as it will become easier for the free metal ions to precipitate under this circumstances. When using in-line UV or ozone in a reciruclating environment it often becomes necessary to be careful with the analysis and replenishing of chelated heavy metals, especially iron.
Plant root system contributions (exudates). The plants will also contribute chemicals to the nutrient solution, which will increase both the carbon content and the biological activity of the nutrient solution. These substances can severely impact the growth and development of the plants as well, as these exudates can contain hormonally active molecules that trigger biological processes within plants. You can eliminate most of these molecules by the use of carbon filters and oxidative sterilization processes but this will cause some of the same issues mentioned in the previous paragraph about pathogen contamination. Carbon filters will also need to be checked and replaced regularly otherwise they will just fill up and become ineffective.
Accumulation of non-nutrient substances. Some ions that are added with water will not be used as nutrients and will just tend to accumulate in a nutrient solution until they become poisonous to plants. The most important accumulation problems are related with sodium and chloride in regions where water contains a significant amount of these ions (like Southern Europe, see here). This is problematic because you will always tend to add these ions with new water additions, so you have limited power to control their accumulation. This might require the use of reverse osmosis systems to add water that contains low levels of these contaminants or – often way more economically – will force the replacement of the solution at some point. Note that poisonous heavy metals – like As, Hg, Cd – can also accumulate with time, reason why the life of a nutrient solution should always be limited, regardless of the efforts made to never replace it. Impurities in your salt inputs can also play an important role in contributing with this non-nutrient accumulations.
I hope the above serves as a good explanation of the common factors that limit the life of a recirculating solution in hydroponics. Maintaining a recirculating nutrient solution is not just “adding water with nutrients to top it off” or “add nutrients to maintain a certain EC”, it requires a substantial amount of care in the evaluation of the nutrient evolution as ions accumulate, other are used and the plants themselves also contribute their own organic molecules to change the makeup of the nutrient solution. In most cases, the solution to just “change the solution every 2 weeks” is just the most economically viable answer but this can be undesirable if both water usage and contamination of water resources wants to be minimized. With good management, solution lifetimes can often be extended to 8-16 weeks, but going beyond that can be risky due to aspects of ion accumulation that are hard to control (as those mentioned in the last problem).