The Potassium to Calcium ratio in hydroponics
To have a healthy hydroponic crop, you need to supply plants with all the nutrients they need. One of the most important variables that determine proper nutrient absorption, is the ratio of Potassium to Calcium in the nutrient solution. These two elements compete between themselves and have different absorption profiles depending on the environment, and the plant species you are growing. For this reason, it is important to pay close attention to this ratio, and how it changes with time, in your nutrient solution. In this post, we are going to examine peer-reviewed research about this ratio and how changing it affects the growth, quality, and yield of different plant species.
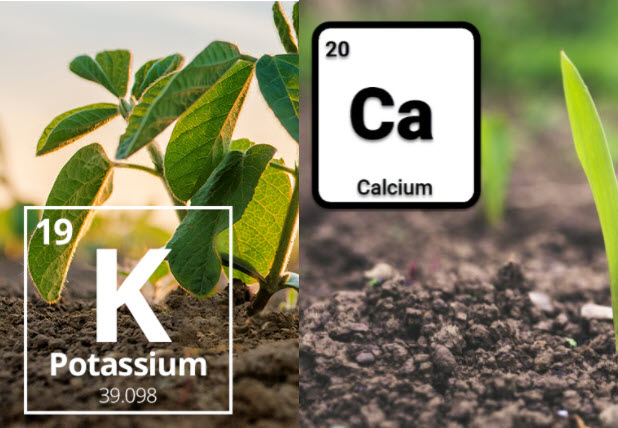
Two ions with very different properties
Potassium and Calcium are very different. Potassium ions have only one positive charge and do not form any insoluble salts with any common anions. On the other hand, calcium ions have two positive charges and form insoluble substances with a large array of anions. This creates several differences in the way plants transport and use these two nutrients.
While potassium is transported easily and in high concentrations through the inside of cells, Calcium needs to be transported in the space between cells and its intracellular concentration needs to be very closely regulated. Calcium can also only be transported up the plant – from roots to shoots – while potassium can be transported up and down as it pleases.
Calcium transport – happening around cells – is heavily dependent on transpiration, which is what causes water to flow through this space. Potassium transport is not so closely related to transpiration, as it can move directly through the inside of cells in large amounts, which means it can be actively transported through the plant in an effective manner.
Note that the above is a broad over-simplification of Potassium and Calcium transport. If you would like to learn more about this topic, I suggest reading these reviews (1,2).
Competition between K and Ca
Potassium and Calcium are both positively charged, so they do compete to a certain extent. The competition is both because they compete for anions – which they need to be paired with for transport – and for the use of electrochemical potential, which they take advantage of to get transported across membranes. However, they do not have the same transport mechanisms, so the competition is limited.
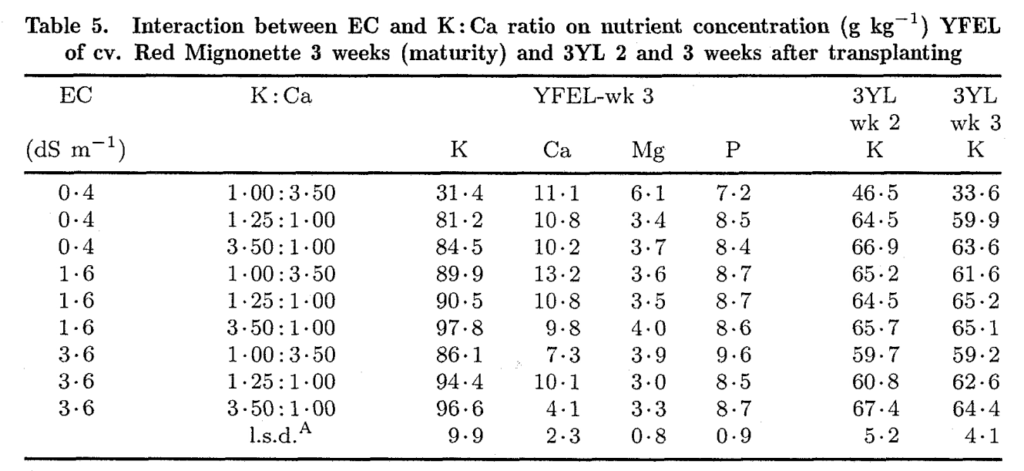
The table above illustrates this point. This study (3) looked into different K:Ca ratios in the growing of lettuce and the effect these ratios had on yield, tip burn, and nutrient concentrations in tissue. You can see that at low total concentrations (0.4 mS/cm EC) the K in tissue is very low when the amount of Ca is high relative to K, while at higher EC values (1.6 mS/cm EC), the K concentration remains basically unaffected, even if the Ca concentration is 3.5 times the K concentration. While Ca competes effectively with K when the absolute concentration of both is low, this competition of Ca becomes quite weak as the concentration of K and Ca increase. At very high concentrations (3.6 mS/cm EC), the potassium does start to heavily outcompete the Ca, especially when the K:Ca ratio is high (3.5x).
The above is also not common to all plants. For some plants, the competition of Ca and K actually reverses compared to the results shown above. However, it is typical for low and high absolute concentration behaviors to be different, and for the influence of K or Ca to become much lower in one of the two cases.
Optimal K:Ca ratios
The K:Ca ratio has been studied for many of the most popularly grown plants in hydroponics. The table below shows you some of these results. It is worth noting, that the results that maximized yields, often did so at a significant compromise. For example, the highest yield for lettuce came at the cost of a significantly higher incidence of inner leaf tip burn. In a similar vein, the highest yields in tomatoes, at a 3:1 ratio, came at the cost of additional blossom end rot problems. This is to say that, although these ratios maximized yields, they often did so with consequences that wouldn’t be acceptable in a commercial setup. For lettuce, 1.25:1 proved to be much more commercially viable, while still giving high yields.
Ref | Plant Specie | Optimal K:Ca |
4 | Rose | 1.5:1 |
5 | Tomato | 3:1 |
6 | Tomato | 1.7:1 |
7 | Marjoram | 0.5:1 |
8 | Strawberry | 1.4:1 |
9 | Cucumber | 1:1 |
10 | Lettuce | 3.5:1 |
You can see in the above results, that fairly high K:Ca ratios are typically required to increase yields. For most of the commercially grown flowering plants studied, it seems that a ratio of 1.5-2.0:1 will maximize yields without generating substantial problems in terms of Ca uptake. As mentioned above, higher K:Ca often push yields further, but with the presence of some Ca transport issues. A notable exception might be cucumber, for which the publication I cited achieved the maximum yield at a ratio of 1:1. However, good results were still achieved for 1.5:1.
Another important point about the ratio is that it is not independent of absolute concentration. As we saw in the previous section, the nature of the competition between K and Ca can change substantially depending on the absolute ion concentrations, so the above ratios must be taken within the context of their absolute concentration. The above ratios are generally given for relatively high EC solutions (1.5-3mS/cm).
Conclusion
The K:Ca ratio is a key property of hydroponic nutrient solutions. While the optimal ratio for a given plant species cannot be known apriori, it is reasonable to assume that the optimal ratio will be between 1:1 and 1:2 for most large fruiting crops and flowering plants that are popularly grown in soilless culture. This is especially the case if the hydroponic solution does not have a low EC. An optimal value below 1:1 is unlikely for most plants, although exceptions do exist in certain plant families that have peculiar Ca metabolisms.
To obtain the largest benefit, it would be advisable to run trials to optimize the K:Ca ratio for your particular crop, by changing the K:Ca ratio between 1:1, 1.5:1, and 2:1. You will likely see important differences when you carry out these trials, which will be useful to determine the highest yielding configuration for your setup. To perform these variations, it is usually easiest to change the ratio of potassium to calcium nitrate used in the nutrient solution.
Have you tried different K:Ca ratios? What do you grow and what has worked for you? Share with us in the comments below!